Hello everyone, this is Yigene, which has been focusing on epi-omics for more than ten years and is a leader in multi-omics research services.
On March 2, 2021, the team of Professor Stacy M. Horner from the Department of Molecular Genetics and Microbiology at Duke University Medical Center published a paper entitled "Post-transcriptional regulation of antiviral gene expression" in the journal "Cell Reports" (IF: 9.995) by N6-methyladenosine” research paper, the study revealed that m6A has an effect on IFN-stimulated genes (IFN-stimulated genes, ISG) Transcriptional regulation mechanism of translation.

标题:Post-transcriptional regulation of antiviral gene expression by N6-methyladenosine
Time: 2021.03.02
Journal: Cell Reports
Impact Factor: IF 9.995
Technology platform: MeRIP-seq, RNA-seq, Ribo-seq, MeRIP-qRT-PCR, luciferase activity analysis experiments, etc.
research summary
Type I interferons (IFNs) respond to viral infection by inducing hundreds of IFN-stimulated genes (ISGs). still uncertain. This study first identified the role of RNA base modification N6-methyladenosine (m6A) in the regulation of ISGs, and identified IFITM1-containing genes by Ribo-seq and quantitative mass spectrometry combined with m6A immunoprecipitation sequencing (MeRIP-seq) analysis. A subset of ISGs whose translation is enhanced by the m6A and m6A methyltransferase proteins METTL3 and METTL14. The study further showed that the m6A recognition protein (readers) YTHDF1 up-regulated the expression of IFITM1 in an m6A binding-dependent manner, and the m6A methyltransferase complex could promote the antiviral activity of type I IFN. In conclusion, this study demonstrates that m6A plays a role in the transcriptional regulation of ISG translation during antiviral-restricted type I IFN responses.
Experimental results
(1) METTL3/14 regulates the translation of certain ISGs
IFN-β forms a response to viral infection by inducing ISG transcription. To investigate whether m6A regulates the type I IFN response, this study examined the IFN-β-induced expression of several ISGs with antiviral functions following deletion of the m6A methyltransferase complex METTL3/14 in Huh7 cells. IFN-β-induced ISG IFITM1 and MX1 protein expression was reduced after METTL3/14 knockout (Fig. 1A), while IFN-β treatment in A549 cells, primary neonatal human dermal fibroblasts (NHDF) and Huh7 cells Similar results were observed at multiple time points (8h, 16h and 24h); however, the effect of MX1 protein levels in A549 and NHDF cells was not as strong as in Huh7 cells. In response to IFN-β, METTL3/14 overexpression increased the abundance of IFITM1 and MX1, but not ISG15 and EIF2AK2, in Huh7 cells (Fig. 1B). The METTL3/14-regulated ISGs IFITM1 and MX1 were not expressed in the absence of IFN-β, suggesting that any confounding effects of METTL3/14 dynamics on endogenous IFN-β were negligible (Figure 1A and 1B).

Figure 1: METTL3/14 regulates the translation of certain ISGs
(AB) METTL3/14 (M3/14) or control (CTRL) were transfected with siRNA before mock or IFN-β (24h) treatment (A) or stably overexpressed FLAG-METTL14 (M3/14OE; upper arrow indicated FLAG-METTL14; down arrow indicates endogenous METTL14) (B) Immunoblot analysis of Huh7 cell extracts. Relative ISG expression of 4 replicates in A and B was quantified relative to a non-targeting control (siCTRL) + IFN-β (A) or WT + IFN- (B).
(CE) Relative percentages of IFITM1 (C), MX1 (D) and GAPDH (E) mRNA in 24 sucrose gradient fractions isolated from extracts of Huh7 cells treated with IFN-β after transfection with CTRL or METTL3/14 siRNA qRT-PCR analysis. Uninitialized (free, 40S and 60S subunits), initialized (80S), low or high molecular weight polysomes. The graph on the right shows the percentage of mRNA in the combined fractions of IFITM1, MX1 or GAPDH. Add the percentages for each section to get the total percentage for each category.
Values are mean ± SEM of 4 biological replicates (A and B), mean ± SD of 3 technical replicates, mean ± SEM of 3 experiments (C–E, left panel) and 3 biological replicates (C–E, right panels). *Unpaired Student's t-test (A and B) and two-way ANOVA with Sidak's multiple comparison test (C–E), *p<0.05, ***p<0.01, ***p<0.005, ns, not significant.
To investigate how METTL3/14 regulates the protein levels of certain ISGs, the study first analyzed whether loss of METTL3/14 resulted in reduced ISG mRNA response to IFN-β. Meanwhile, using RNA sequencing (RNA-seq) after IFN-β treatment, the results showed that METTL3/14 deletion had little effect on the mRNA expression abundance of core ISGs, suggesting that ISG RNA stability was not affected by METTL3 deletion.
Since deletion of METTL3/14 resulted in decreased IFITM1 and MX1 proteins without affecting their transcription levels, this study analyzed whether METTL3/14 regulates their protein stability. The findings suggest that METTL3/14 cannot detect nuclear export or protein stability of these ISGs in Huh7 cells.
To test whether METTL3/14 regulates IFITM1 translation, the authors measured the percentage of polysomes in control cells or in METTL3/14-depleted cells after IFN-β treatment. The results showed that METTL3/14 deletion did not change the total polysome level, but METTL3/14 deletion resulted in lower IFITM1 mRNA levels in the 80S fraction and a shift from heavy polysome fraction to light polysome fraction (Fig. 1C) , indicating that IFITM1 translation is impaired following METTL3/14 deletion. Additionally, similar but less pronounced changes were observed in MX1 (Fig. 1D) but not in GAPDH (Fig. 1E). Taken together, the results suggest that METTL3/14 regulates the translation of certain ISGs, such as IFITM1 and MX1.
(2) ISGs regulated by METTL3/14 are modified by m6A
To determine whether the METTL3/14-regulated ISGs IFITM1 and MX1, as well as other ISGs, are modified by m6A, we mapped IFN-induced transcriptome m6A in Huh7 cells by using methylated RNA immunoprecipitation sequencing (MeRIP-seq). After ISG, the peaks of reads coverage after m6A immunoprecipitation were compared with the input using MeTDiff m6A peaks calling. The results showed that mRNA peaks were enriched at the ends of coding sequences and 3′UTR start sites (Fig. 2A). The most enriched RNA sequence motif in peaks is [U/A]GGAC, which matches the known m6A motif of DRAmC (Fig. 2A). About 85% of ISGs (ISGs classified as upregulated more than 4-fold after IFN) were modified by m6A, while 74% of Huh7 cells expressed transcriptome (mean coverage ≥10) (Fig. 2B). This result is consistent with a previous study that found that ISGs undergo m6A modification at a similar percentage to the transcriptome. The percentage of m6A-modified ISGs was similar in other classes of ISGs, including the evolutionarily conserved "core" ISGs in vertebrates and 14 subpopulations of core ISGs with known antiviral functions (Fig. 2B). Next, using MeRIP-seq data to generate IFITM1, MX1, ISG15 and EIF2AK2 maps, and using m6A peaks calling methods MeTDiff and meRIPPer revealed that METTL3/14 regulatory genes IFITM1 and MX1 have m6A peaks (Figure 2C and 2D), while ISG15 and EIF2AK2 has no m6A peaks (Fig. 2E and 2F), and these figures suggest that the 3'UTR of ISG15 may also contain m6A sites (Fig. 2E). Comparing the m6A status of ISGs in MeRIP-seq experiments with published study data, the comparison results showed that the m6A status prediction of core antiviral ISGs was consistent in all studies. dsDNA treatment efficiently activated IFN production and induced m6A modification of the same core antiviral ISGs found in this experiment. HCMV infection also results in m6A modification of certain ISGs, which encode factors that inhibit IFN signaling; thus ISGs may not be as strongly induced by dsDNA or IFN-β treatment. The presence of m6A on many ISGs suggests that m6A can modulate antiviral type I IFN responses.
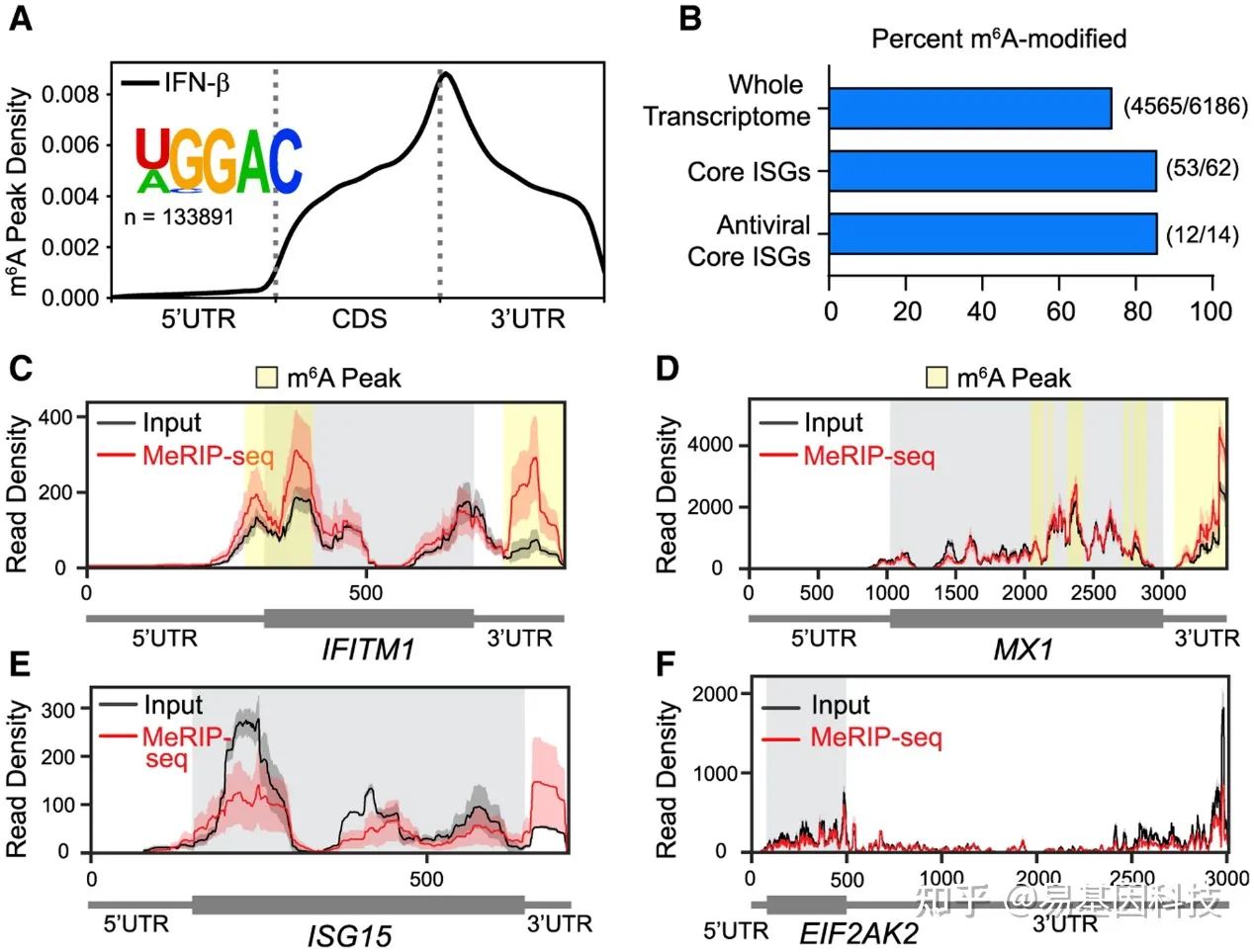
Figure 2: METTL3/14-regulated ISGs are modified by m6A
(A) Transcriptome-wide m6A distribution metagene map after IFN-β treatment (8h), plotting the relative position of DRACH motif sites in statistically significant peaks, and the most enriched motif in peaks.
(B) Percentage of genes modified by m6A in the expressed transcriptome, genes induced ≥4-fold by mRNA in response to IFN-β treatment (ISG), core ISGs conserved in vertebrates, or core ISGs with antiviral function subgroup.
(CF) Coverage of MeRIP (red) and input (black) reads in IFITM1 (C), MX1 (D), ISG15 (E), and EIF2AK2 (F) transcripts. Differences in biological replicates are indicated by red and black shading around read coverage, gray shading indicates coding sequences, and yellow shading indicates m6A peaks calling software by MeTDiff and meRIPPer. All analyzes were performed on 3 biological replicates.
(3) The m6A modification of IFITM1 in the 3′UTR enhances its translation
m6A enhances the translation of certain mRNAs. Specifically, the m6A recognition protein (readers) YTHDF1 recognizes m6A within the 3′UTR and binds to eukaryotic translation initiation factors such as eIF3 to enhance the translation of m6A modified transcripts. Whether METTL3/14's translational regulation of ISGs is induced by m6A was investigated using IFITM1 as a METTL3/14-regulated ISG model. We first determined the effect of METTL3/14 deletion on IFITM1 m6A modification. MeRIP-qRT-PCR results showed that IFITM1 mRNA was enriched in m6A-negative ISG EIF2AK2 and m6A-negative synthetic RNA, confirming that it contained m6A. Deletion of METTL3/14 reduced m6A enrichment of IFITM1 mRNA, but not m6A-negative EIF2AK2 transcripts or m6A-negative synthetic RNA (Figures 3A and 3B), data suggesting that IFITM1 is m6A modified by METTL3/14.
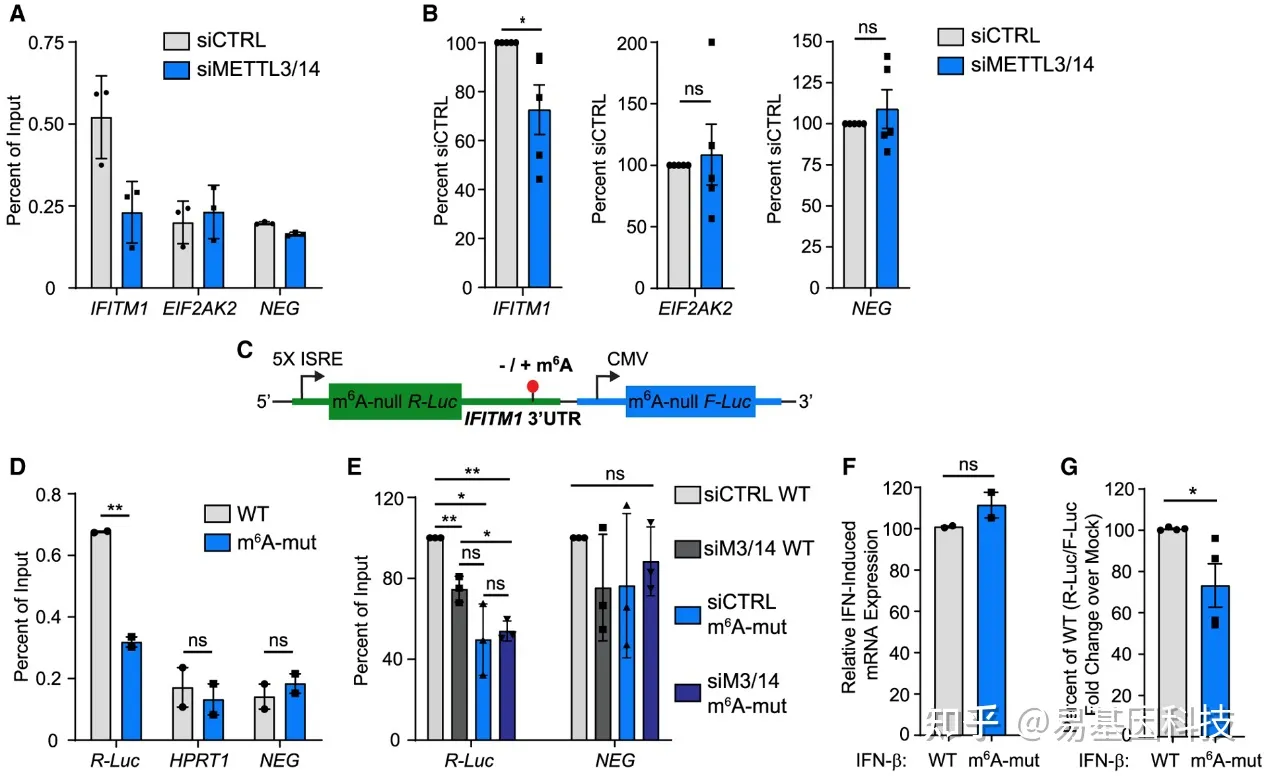
Figure 3: m6A modification of IFITM1 in the 3'UTR enhances translation
(A) Representative MeRIP qRT-PCR of relative mA levels of ISGs induced by IFN-β (8 h) in Huh7 cells treated with siCTRL or METTL3/14 siRNA and incorporated with m6A-negative (NEG) oligonucleotides analyze.
(B) Relative percent enrichment for each gene of the 5 biological replicates in A, normalized to siCTRL.
(C) Schematic of the wild-type (WT) and mutant ISRE-m6A-deleted Renilla luciferase (R-Luc) IFITM1 3'UTR reporter gene, which also expresses m6A-deleted firefly luciferase from a separate promoter. (F-Luc).
(D) MeRIP-qRT-PCR analysis of relative mA levels of WT and m6A-mut IFITM1 3'UTR reporter RNA of transfected Huh7 cells treated with IFN-β (8 h).
(E) MeRIP of relative mA levels of WT and m6A-mut IFITM1 3'UTR reporter RNA transfected with siRNA (24h), followed by reporter gene transfection (24h) and treated with IFN-β (8h). -qRT-PCR analysis,.
(F) qRT-PCR analysis of WT and m6A-mut IFITM1 3′UTR reporter mRNA expression normalized to HPRT1 in Huh7 cells after reporter transfection (24 h) and IFN-β treatment (8 h).
(G) Relative luciferase activity (R-Luc/F-Luc) in IFN-β-induced WT and m6A-mut IFITM1 3′UTR reporter genes (relative to mock 8h).
After identifying IFITM1 as an m6A modification, a luciferase reporter gene containing an IFN-stimulated response element (ISRE)-promoter driven Renilla luciferase was next generated with all DRAC motifs knocked out (m6A- null R-Luc), then combined with wild-type (WT) IFITM1 3′UTR or similar 3′UTR sequence, four putative m6A motifs in the 3′UTR m6A peaks in IFITM1 switched from A→G (m6A-mut) (Fig. 3C). Analysis of the data revealed that METTL3/14 regulated IFITM1 translation by adding m6A modification to the 3'UTR, and m6A modification within the 3'UTR of IFITM1 was sufficient to enhance its translation.
(4) YTHDF1 enhances IFITM1 protein expression in an m6A-dependent manner
To test whether YTHDF1 induces the translational promotion effect of m6A on ISGs, the researchers stably overexpressed YTHDF1 (H7Y1) or m6A-binding-deficient YTHDF1 protein (H7Y1mut) in Huh7 cells and analyzed their expression relative to parental Huh7 cells (H7) after 24 h. IFN-induced ISG expression. YTHDF1 overexpression increased IFITM1 protein expression in response to IFN-β, whereas overexpression of mA binding-deficient YTHDF1 protein (H7Y1mut) did not increase IFITM1 abundance (Figures 4A and 4B). Moreover, wild-type (WT) and mutant YTHDF1 overexpression did not significantly affect IFITM1 mRNA levels after IFN-β treatment, suggesting that YTHDF1 does not directly regulate IFN signaling or IFITM1 mRNA stability (Fig. 4C). YTHDF1 overexpression significantly altered the IFN-induced expression of the m6A-containing ISG MX1, or the m6A-free ISG ISG15 and EIF2AK2 (Fig. 4A and 4B). After IFN-β treatment, loss of YTHDF1 resulted in decreased protein expression of IFITM1, but MX1, ISG15, and EIF2AK2 were not affected (Fig. 4D). Additionally, WT YTHDF1 bound transcripts from IFITM1, MX1, ISG15, and m6A-positive control SON, whereas m6A-binding-deficient YTHDF1 muteins did not. The mRNAs EIF2AK2 and RPL30 containing non-m6A did not bind to either protein (Fig. 4E and 4F). Taken together, these results suggest that YTHDF1 binds to mA on IFITM1 mRNA and enhances its translation through its mA-binding activity is necessary and sufficient. The apparent m6A-dependent binding of YTHDF1 to ISG15 mRNA suggests that ISG15 mRNA is actually m6A modified. The association map of input reads and MeRIP-seq reads in ISG15 mRNA revealed potential regions of m6A enrichment in its 3'UTR (Fig. 2E). Thus, YTHDF1 has a transcript-specific role in promoting translation because it binds transcripts of IFITM1, MX1, and ISG15, but its overexpression is only sufficient to significantly increase IFITM1 protein production.
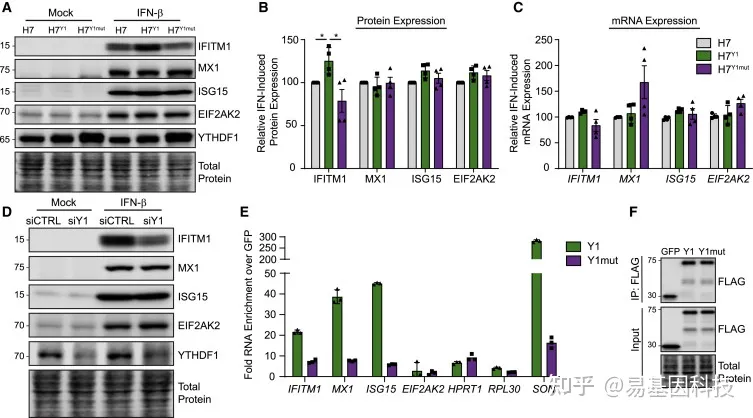
Figure 4: YTHDF1 enhances IFITM1 protein expression in an m6A-dependent manner
(A) Immunoblot analysis of extracts from Huh7 cells stably overexpressing FLAG-YTHDF1 WT (Huh7Y1) or FLAG-YTHDF1 W465A (Huh7Y1mut) after mock or IFN-β (24 h) treatment.
(B) Quantification of ISG expression after IFN-β treatment from 3 independent experiments in A, normalized to total protein and plotted relative to siCTRL.
(C) qRT-PCR analysis of ISG mRNA expression normalized to HPRT1 in Huh7 cells stably overexpressing FLAG-YTHDF1 WT (Huh7Y1) or W465A (Huh7Y1mut) after IFN-β (24 h) treatment.
(D) Immunoblot analysis of extracts from Huh7 cells transfected with siRNA to YTHDF1 (siY1) or siCTRL before mock or IFN-β (24 h) treatment. Data are representative of 3 independent biological experiments.
(E) qRT-PCR analysis of mRNA enrichment after immunoprecipitation (IP) of FLAG-YTHDF1 WT (Y1) or W465A (Y1mut) compared with FLAG-GFP of Huh7 cells after IFN-β (8h) treatment. IP values were normalized to input values and plotted as fold enrichment for GFP.
(F) Immunoblot analysis of FLAG immunoprecipitated and input fractions used in E.
(5) METTL3/14 and m6A promote the translation of ISG subsets
To identify other ISGs whose protein expression is regulated by METTL3/14, quantitative mass spectrometry-based proteomics and stable isotope labeling of amino acids (SILAC) were used to compare the proteomes of siCTRL and siMETTL3/14 cells after IFN-β treatment. Compared with siCTRL, the effect of siMETTL3/14 on protein abundance was concentrated in most proteins with a log ratio of 0, indicating that METTL3/14 deletion had no overall effect on protein levels after IFN-β treatment. In previous RNA-seq experiments, ISGs were defined as genes upregulated >2-fold by IFN-β treatment to determine which proteins were ISGs. Despite limited detection of ISGs by mass spectrometry (n = 18), some METTL3/14-regulated ISGs were identified (Fig. 5A; MS). The protein expression of most of these ISGs is reduced after METTL3/14 deletion, and these ISGs include the previously identified m6A-modified IFITM1 (corresponding to the peptide of IFITM1/2/3) and MX1, as well as other antiviral ISGs such as OAS2 and different HLA-C chain (Fig. 5A), all modified by m6A. By comparing these data with those from previous RNA-seq experiments, it was shown that the effect of METTL3/14 on the protein levels of these ISGs was not determined by regulation of their mRNA expression, as METTL3/14 depletion was reduced at the protein level in this experiment The ISG mRNA abundance was not reduced, suggesting translational regulation consistent with previous polysome analysis of IFITM1 and MX1 (Figure 1C and 1D; Figure 5A, RNA). Not all m6A-modified ISGs identified by mass spectrometry were regulated by METTL3/14 loss (Fig. 5A; m6A), suggesting that METTL3/14 and m6A regulate a subset of ISGs and support their protein expression.
Figure 5: METTL3/14 regulates translation of ISG subsets
(A) 3-column heatmap showing the effect of METTL3/14 deletion on ISG expression in Huh7 cells after IFN-β treatment. The first column shows the log2 fold change in estimated protein detection by quantitative mass spectrometry (siMETTL3/14 vs. siCTRL + IFN-β 24 h; n = 2 biological replicates). The second column shows the log2 fold change in mRNA reads from independent RNA-seq experiments (siMETTL3/14 vs. siCTRL + IFN-β 8 h; n = 3 biological replicates). The third column shows the m6A status of the MeRIP-seq data (+ means m6A positive; - means m6A negative) (+IFN-β 8h; n=3 biological replicates). Genes including any ISGs induced more than 2-fold by IFN by RNA-seq that were also detectable by mass spectrometry are shown in bold in other panels. Since IFITM1/2/3 are similar, this notation is used to denote peptides detected from this protein family; RNA-seq fold change and m6A status correspond to underlined numbers, adjusted *p<0.05.
(B) Four-quadrant scatter plot of the effect of METTL3/14 deletion on ISG expression. The y-axis is the log2-fold change in ribosome-protected fragments from Ribo-seq (siMETTL3/14 over siCTRL), and the x-axis is the log2-fold change in mRNA reads from independent RNA-seq experiments (siMETTL3/14 vs. siCTRL). m6A-modified (blue) or m6A-negative (gray) genes. ISGs in other figures are labeled.
(6) METTL3/14 enhances the antiviral effect of IFN response
METTL3/14 enhances ISG subset expression during type I IFN responses, suggesting that it may be required for optimal antiviral The researchers examined the ability of type I IFN to restrict infection by the negative-sense RNA virus vesicular stomatitis virus (VSV) following dynamic changes in METTL3/14. The VSV genome contains the m6Am cap modification, but since this modification deposition is not regulated by METTL3/14, VSV replication may not be directly affected by METTL3/14 dynamics, whereas any effect on VSV replication may be determined by the expression of host transcripts. Methylation-mediated. METTL3/14 expression was disrupted by using small interfering RNA (siRNA) or overexpression, then examined 6 hours post-infection using microscopy in the presence and absence of low-dose IFN-β pretreatment (6h; 40U/mL) Percentage of cells infected by VSV. Detection of VSV infection at early post-infection time points allowed the detection of viral replication prior to the upregulation of ISG cells induced directly by infection. The results showed that no ISG induction by VSV was seen under any condition without IFN-β pretreatment (Fig. 6A and 6B). VSV replication, detected by immunoblotting or quantitative analysis of percent infected cells, was unchanged in the absence or overexpression of METTL3/14 in the absence of IFN-β treatment (Fig. 6). After IFN-β pretreatment, METTL3/14 deletion resulted in decreased expression of METTL3/14-regulated ISGs (Fig. 6A), whereas METTL3/14 overexpression upregulated its expression (Fig. 6B). Although IFN-β pretreatment reduced VSV viral replication, METTL3/14 deletion reduced the ability of IFN-β to restrict VSV, whereas METTL3/14 overexpression enhanced IFN-mediated VSV restriction (Fig. 6). Together these data suggest that METTL3/14 enhances the antiviral properties of type I IFNs and is required for efficient IFN-mediated antiviral responses.
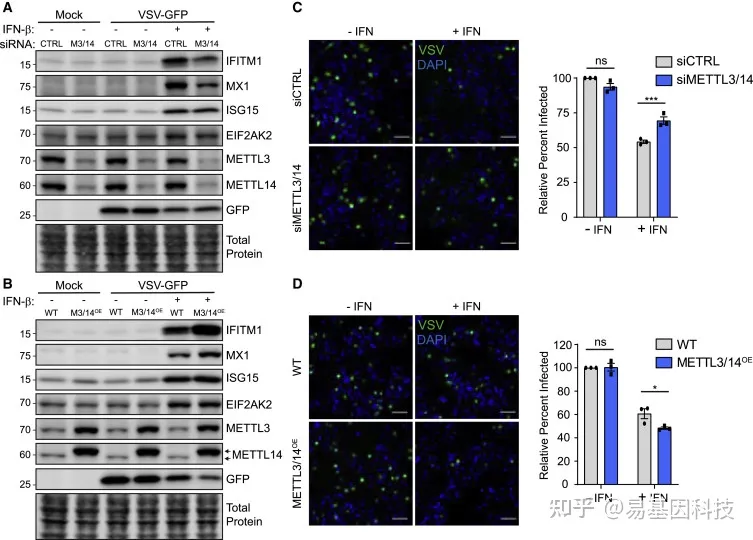
Figure 6: METTL3/14 enhances the antiviral effect of type I IFN responses
(AB) Representative immunoblot analysis (n=3) of Huh7 cell extracts transfected with siRNA (A) or stably overexpressing FLAG-METTL14, which also enhanced METTL3 expression (M3/14OE); 6h) or mock treatment, followed by VSV infection (MOI = 2; 6h) (B). Arrows indicate FLAG-METTL14 (upper) and endogenous METLL14 (lower).
(CD) Representative micrographs of Huh7 cells treated with siCTRL or METTL3/14 siRNA (C) or stably overexpressing FLAG-METTL14 (METTL3/14OE; D), which were pretreated with IFN-β (6h), VSV infection was then performed (MOI=2; 6h) and the percentage of cells infected was quantified from 3 independent experiments with 5 fields per condition, >150 cells per field, normalized to siCTRL without IFN treatment or WT, as shown on the right. Scale bar: 100 μm.
About Yigene RNA m6A Methylation Sequencing (MeRIP-seq) Technology
Yigene MeRIP-seq technology uses m6A-specific antibodies to enrich m6A-modified RNA fragments (including mRNA, lncRNA and other rRNA to remove all RNA), combined with high-throughput sequencing, it can locate and quantify the m6A modification on RNA. The input amount of RNA can be reduced to 10 μg, and only 1 μg of total RNA is required. Widely used in tissue development, stem cell self-renewal and differentiation, heat shock or DNA damage response, cancer occurrence and development, drug response and other research fields; it can be applied to m6A detection of animals, plants, cells and tissues.
For association analysis of m6A-QTL traits with a large sample size, the price of a single sample of traditional MeRIP is high, which is usually unaffordable. Yigene developed and established the MeRIP-seq2 technology, which significantly increases the parallelism of IP, realizes relative quantification among different samples, and reduces detection costs.
Yigene provides MeRIP technology suitable for different scientific research needs:
- m6A methylation - constant mRNA methylation sequencing (MeRIP-seq)
- m6A methylation - constant mRNA + lncRNA methylation sequencing (lnc-MeRIP-seq)
- m6A methylation-micro mRNA + lncRNA methylation sequencing (Micro-lnc-MeRIP-seq)
- High-throughput m6A methylation-constant mRNA methylation sequencing (MeRIP-seq2)
Technical advantages:
- Low input amount: the sample input amount can be reduced to 10-20μg, and the minimum requirement is only 1μg total RNA;
- Transcriptome-wide: mRNA and lncRNA can be detected simultaneously;
- Sample requirements: It can be used for m6A detection of animals, plants, cells and tissues;
- High repeatability: IP enrichment has high repeatability, minimizing the deviation of antibody enrichment;
- Wide range of applications: Widely used in tissue development, stem cell self-renewal and differentiation, heat shock or DNA damage response, cancer occurrence and development, drug response and other research fields.
research direction:
m6A methylation is currently mainly used in theoretical research on molecular mechanisms
- Disease development: tumors, metabolic diseases (e.g. obesity/diabetes), neurological and psychiatric diseases (e.g. Alzheimer's disease/depression), inflammation…
- Development and differentiation: early embryonic development, individual/tissue/organ growth and development, stem cell differentiation and fate determination, aging
- Environmental Exposure and Response: Pollution, Stress Resilience, Lifestyle
About m6A methylation research ideas
(1) Grasp the characteristics of the m6A methylation map as a whole: changes in the number of m6A peaks, changes in the number of m6A modified genes, analysis of the number of m6A peaks in a single gene, distribution of m6A peaks on gene elements, motif analysis of m6A peaks, and changes in the number of m6A peak modified genes Functional Analysis
(2) Screen specific differential m6A peaks and genes: identification of differential m6A peaks, analysis strategies for non-sequential data, analysis strategies for time-series data, functional analysis of differential m6A modified genes, PPI analysis of differential m6A modified genes, m6A modification of candidate genes Visualization
(3) Association analysis of m6A methylomics & transcriptomics: overall association of Meta genes, DMG-DEG correspondence association, screening strategy for m6A modified target genes
(4) Further verification or post-test

Yigene Technology provides a comprehensive overall solution for RNA methylation research.

references:
McFadden MJ, McIntyre ABR, Mourelatos H, Abell NS, Gokhale NS, Ipas H, Xhemalçe B, Mason CE, Horner SM. Post-transcriptional regulation of antiviral gene expression by N6-methyladenosine. Cell Rep. 2021 Mar 2;34(9):108798.
Related Reading:
Dry goods series: data mining ideas for m6A RNA methylation research
Dry goods series: preliminary exploratory experimental ideas for m6A RNA methylation research